After writing my last post on why humans keep getting deadly viruses from bats, I got some interesting questions on why some viruses cross over from animals but most do not. What needs to happen for a virus to start infecting humans? Or, perhaps more pertinently, what new viruses do we need to worry about next?
The short answer is that a lot needs to happen for a virus to successfully infect humans. It needs to survive in human bodies, not get taken out by human immune systems, successfully infect human cells, then successfully pass from one human to the next.
This is a difficult enough task that the only viruses that we probably need to worry about are the ones that have successfully crossed over to humans in the past. Bird flu caused pandemics in 1918 (probably), 1957, and 1968. It’s likely that it’ll do so again. On the other hand, it is unlikely that a virus with no history of crossing over to humans can mutate enough to successfully infect humans.
That’s the short answer. The long answer is a lot more complicated.
Before I start, it’s important to note that virology is still in its infancy. No scientist could design a virus from scratch to successfully infect humans, or even predict in advance whether a virus can infect humans. Virologists are still at the point where they’re only collecting data on which viruses have successfully infected humans and how they’ve done so.
As such, our answers as to how viruses are spread to humans are still frustratingly specific. We’re going to have to look at a case study, H5N1 (the most recent bird flu), and ask the question: how could H5N1 spread to humans?
H5N1 was a flu that seemed prime to spread to humans. It successfully crossed over a few times in poultry markets and environmentally, although not as often as one would expect considering how widespread it was among ducks, geese, chicken, and other farm birds. It was also related to the bird flus that did successfully spread to humans in 1957 and 1968. Finally, the relatively few times it did cross over, it killed people very effectively, reaching a mortality rate of about 60%.
So what were those extra steps that H5N1 needed to take? How did it need to change to successfully spread from person to person?
Well, let’s start by looking at it in the wild, or what’s called its wildtype. Then we can look at how it had to change.
H5N1 is 8 strands of RNA (which humans use for messaging DNA info), wrapped in a lipid coat, studded with the proteins NA, HA, and M2 (note: the entire coat is studded with these proteins, they just drew only 3 of them).
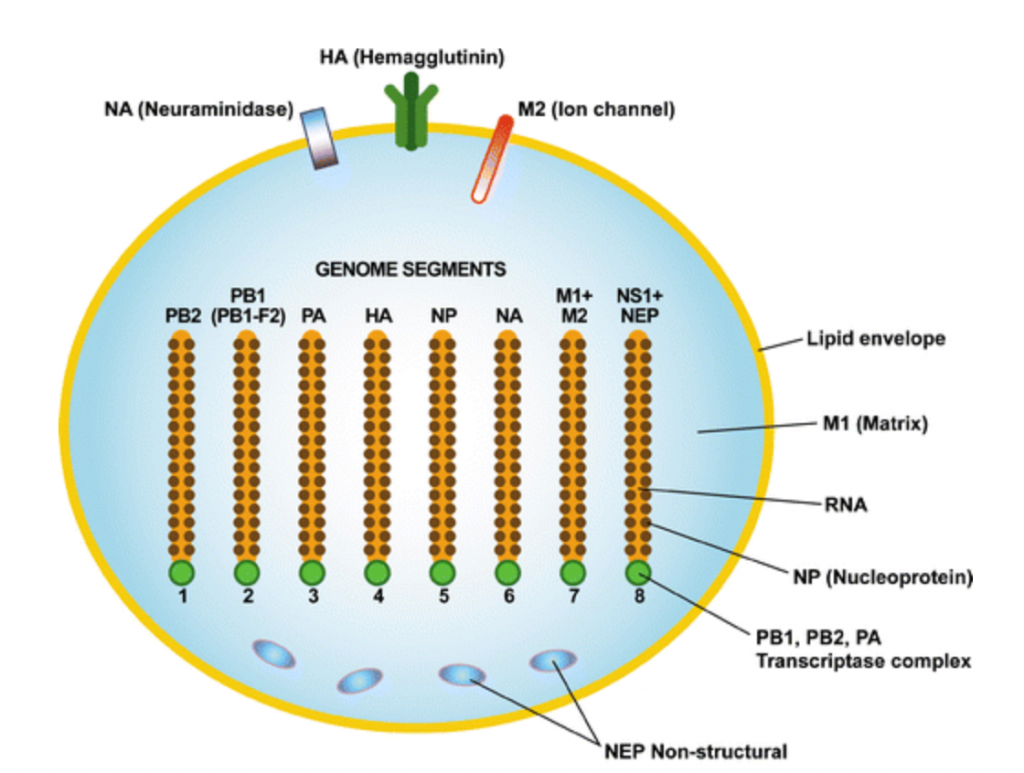
This is an incredibly simple and tiny setup. It’s way smaller and simpler than a cell. There’s a good reason for that: a cell has to have all the machinery to run. A virus just needs to hijack the cell’s machinery: it’s a strike team, as opposed to a factory.
H5N1’s ultimate goal is to get its instructions running in the cell. In order to do so, it first needs to actually survive in a bird’s body.
Then, the virus needs to evade the body’s innate immunity, like the mucus that lines the respiratory tract, the macrophages that eat up anything unfamiliar, and the tough skin cells that don’t let anything through.
Next, it needs to evade the body’s adaptive immunity, made up of antibodies looking to spot viruses that it’s encountered before. The most recognizable parts of the H5N1 virus are the relatively large HA and NA proteins, followed by the relatively small M2 ion channel. Influenza A viruses all have these features, so, generally speaking, antibodies are on the lookout for them. They might not have seen H5N1 before, but they’ve seen its cousins.
To respond to this, influenza A viruses like H5N1 constantly change around the shape and structure of their HA and NA proteins to make it harder for the antibodies that are trying to spot them. This is, of course, a random process, driven by viruses’ constant replication and poor error-checking of their RNA build-out instructions. Any mutations that are beneficial will make that virus type way more common (more of them will survive). This is a balancing act, though: HA and NA need to change enough to be unrecognizable, but they do still need to be functional.
If H5N1 does evade the immune system, it then needs to find an appropriate cell. When it does, the virus uses HA to attach itself to sialic acid residues on the outside of the cell. Like all protein-protein interaction, this is really specific and works on a lock and key method.
In fact, it’s so specific that the best theory on why H5N1 could not infect humans at first was that HA needed to be modified to attach to a different side of the same acid of the same protein that humans and birds both share. It’s like a lock that not only just accepts one key, but only accepts a key that’s bent in exactly the right way, and viruses have to accidentally bend their key in the right way to fit.
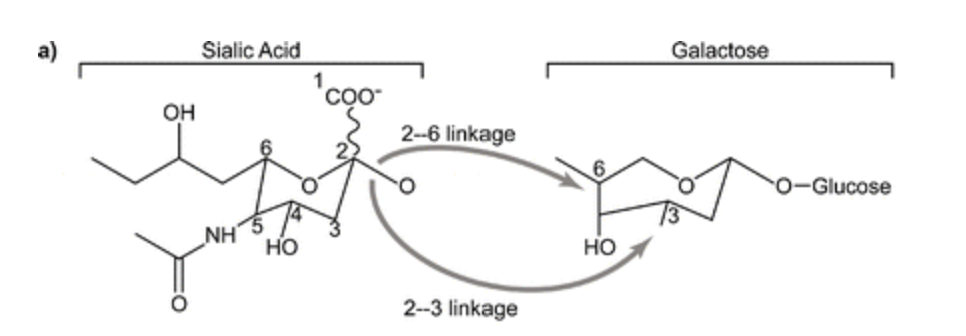
On a side note, this is where NA comes in handy, too. HA not only only attaches to very specific protein residues, but it also doesn’t care where those protein residues are. This is a problem for the virus if those protein residues are in mucus, which has a lot of sialic acid but no viable cells to infect. NA serves to bushwack the virus’s way through decoys until it can get to the real prize.
After the virus attaches to a cell, it works its way to an endosomal compartment, which is a gateway into the cell. Normally, it’s difficult for viruses to pass through this gateway, for obvious reasons.
However, H5N1 has a trick. It found a way to use proteases (the body’s tools to break down and manipulate proteins) to transform the HA protein into two proteins that can fuse with the endosomal gateway, allowing the virus to enter. Proteases, like proteins, are really specific, and this trick only works on proteases that are found in the respiratory and gastrointestinal tract (which is why avian flu causes pneumonia and diarrhea).
Then, it uses M2 as a channel to inject its RNA into the cell. From there, the virus uses its own polymerases to make more copies of its RNA, so it can start taking over the cell.
Finally, it needs to spread from human to human, like through a cough or a sneeze. This is a complex process that’s not super well understood, but likely requires high levels of virus in the upper respiratory tract. This results in inflammation, which then results in coughing or sneezing.
The main barriers to wildtype H5N1’s success came with attaching to cells (as previously mentioned), making more copies of its RNA in the different temperature and pH of the human body, and making humans cough or sneeze. So, in order for H5N1 to successfully transmit from human to human, it’d probably need to figure that out.
Fortunately for us, we have experimental confirmation on exactly how that would happen, with the really interesting and kind of frightening paper “Airborne Transmission of Influenza A/H5N1 Virus Between Ferrets“. In this paper, they turned H5N1 into a virus that could probably be transmitted from human-to-human using ferrets as a vector.
Why ferrets? Well, because ferrets can be infected by both human and avian flus. When ferrets get human flus, they react to them much like humans would, and can spread them to each other. They’re an ideal mixing bowl, like pigs are, but a lot easier to keep.
In order for H5N1 to be spread between ferrets, there were a few changes that needed to be made. Two of them they knew about, and did so in the experiment by directly editing the viral genome to change the protein HA and the viral polymerase PB2:
- Making sure that HA in H5N1 bonded to 2,6 sialic acid instead of 2,3
- Changing PB2 to replicate more efficiently at the lower temperature of the mammalian respiratory tract (33 degrees C) rather than the higher temperature of the avian respiratory tract
However, once they made those changes (which, for the record, can be done with off the shelf parts), they found that the virus still did not transmit between ferrets. So, they decided to introduce artificial selection into the process, and let guided evolution find the missing piece.
They started inoculating the ferrets intranasally with the mutated H5N1 (literally squirting it in the ferrets’ noses). They would inoculate one ferret, let the virus work for a bit, then euthanize the ferret, extract the mutated virus from the now deceased ferret’s lungs and nose, blended all the extract together, and then inoculated a new ferret.
In this process, they were essentially forcefully recombining the viruses again and again. Each time they blended the extract together, the viruses had a chance to swap parts, so whatever mutations one virus had could be shared with the other viruses. Any mutation that caused a virus to be particularly successful (i.e. be more prevalent in the lungs or nose) would be shared with its counterparts.
They did this 6 times, leaving 12 dead ferrets in their wake (their control group was doing the same thing with wildtype virus). Then, they changed tactics, and, after inoculating, didn’t extract the virus directly from the ferrets’ nose and lungs. Instead, they squirted the ferrets’ noses with water and forced them to sneeze, then collected their sneeze. Then, same process: blend the sneeze, inoculate the next ferret with the sneeze, euthanize the old one.
While before they were selecting for any mutation that would cause a virus to be more prevalent in the lungs or nose, in this case they were selecting for mutations that would cause a virus to be more prevalent in sneezes. Any mutation that allowed a virus to be common in sneezes would be shared with its counterparts.
They did that an additional 3 times, leaving 20 dead ferrets in their wake. By this point, they had their virus, and sequenced it. As one might expect, there were a lot of different mutations in the flu virus, which varied from virus to virus, even within the last ferret. But, there was one mutation that almost all the viruses shared: a mutation that removed a piece of HA. As it turned out in a subsequent experiment, this mutation allows HA to fuse with cell membranes at lower pHs (i.e. the pH of human respiratory tracts, rather than avian).

All that was left to do now was test their new, artificially selected virus. And it worked! It successfully spread from ferret to ferret through the air when they were kept in cages next to each other.
The mutated virus had a weird effect when it came to virulence, though: the mutated virus was as deadly as the wildtype intratracheally, less deadly intranasally, and supposedly not deadly at all when transmitted through the air. I say supposedly because they stopped keeping track of the ferrets’ health 3 days after they got infected, which seems way too short to me.
The scientists were also eager to point out that the mutated virus was sensitive to the flu drug oseltamivir, and that it wouldn’t affect people who had antibodies against other H5 flus. My only guess as to why they pointed that out, and why they stopped tracking ferret health after day 3, was that they wanted to forestall criticism about having created a pandemic in a laboratory. They also spent a large amount of their paper talking about their security protocols, which seemed to be for the same purpose.
Regardless, this experiment showed, in a limited way, how viruses can go from animals to humans. It’s not easy: the wildtype virus never became transmissible from ferret to ferret, even after going through the artificial selection process. And it is limited: H5N1 was already mediocre at going from birds to humans, it just wasn’t particularly good at it or at transmitting from human to human.
There’s still a lot of work to be done on viruses. For one thing, scientists still have no ability to predict which mutations a virus needs to undergo in order to transmit to humans. We can observe what happens naturally and copy it, but we can’t predict it.
For another, there’s still not great information on what makes a particular virus so deadly, and how that connects with how well it spreads. There’s some obvious connection, namely that a virus that kills ferrets or humans in 3 days (like H5N1) will result in pretty quick social isolation. But whether making H5N1 airborne actually made it less deadly is a much more interesting and complicated question.
Additional research on predicting how deadly viruses will be humans is complicated, for obvious reasons. Even this paper was really controversial. I think the best avenue of exploration would be predicting how deadly a created virus would be in birds, fish, or insects. There’s still so much to discover on how viruses work on literally any level, so there’s no reason to limit the discovery to humans.
another informative article; well written, easy to understand
Great essay Trevor – please keep them coming.