This is a post I wrote outside my comfort zone, mainly because I was having serious writer’s block about writing things inside my comfort zone. I think everything I wrote is correct, but who am I to judge?
Because there’s literally nothing else interesting or important going on in the world right now, I thought I’d take a close look at this neat paper on bees called Engineered symbionts activate honey bee immunity and limit pathogens and its supplement.
In this paper, they detail how they genetically engineered the gut bacteria of bees to produce double stranded RNA, which they used to cause bees to gain weight, defend themselves against the deformed wing viruses, and kill parasitic Varroa mites. The latter two are the main causes of colony collapse disorder, if you’re familiar.
This is cool already, but the way they did it was cool, too: they figured out what part of the bee/virus/mite genome they needed to target, bought online the custom-made plasmids (small DNA sequences) to produce the RNA that’d target the necessary parts of the genome, put the plasmids into bee gut bacteria, then put the gut bacteria into the bees.
That’s really cool, right? We now live in an era where you can just like… genetically engineer bees with stuff you order online. Realistically, you could genetically engineer yourself with stuff you order online. You could make yourself resistant to a virus or lactose intolerant (if you really wanted to).
Before we wax too rhapsodic, though, let’s talk a bit about exactly what they did, how they did it, what the limitations are, some unanswered questions/issues, and then, finally, how soon this stuff can be actually ready to be live.
So, to explain what they did, let’s start with their goals. Their goal was to cause RNA interference with other RNA strands in the bees’ bodies. RNA, as you might recall from biology class, is a lot like DNA, in that it contains instructions for other parts of the cell. Bees’ bodies (and our bodies) use RNA to help transmit instructions from DNA to cell machinery, while viruses just keep all their instructions in RNA in the first place.
RNA interference, therefore, means that the instructions are being disrupted. If you disrupt the instructions to reproduce a virus, the virus will not be reproduced. If you disrupt instructions to produce insulin, the insulin will not be produced. One of the ways RNA can be interfered with (and the way that these people specifically interfered with RNA) is by double stranded RNA.
Double stranded RNA (dsRNA) is what it sounds like: RNA, but double stranded. This is weird because RNA is usually single stranded. When you put targeted double stranded RNA into the body, an enzyme called dicer dices it (great name, right?) into two single strands.
One of these strands will then be complementary (fits like a puzzle piece) to the target RNA, so it’ll latch onto it, serving as a sort of flag to the immune system. Then a protein called argonaute, now that it knows what it’s targeting, comes in and slices the target RNA in two. The target RNA is effectively interfered with.
Now, this is something that happens naturally in the body all the time as part of the immune system. However, the body has to be producing the right double stranded RNA already, so it can flag things correctly (the flags are super specific). What if the body isn’t producing the right dsRNA yet?
Well, if it isn’t, you need to get the dsRNA in there somehow, so the flagging process can start. One option is, of course, just to inject a ton of double stranded RNA into the body. You have to make it all first, of course, and it has a limited shelf-life, but it’s doable. That’s been done before with bees.
This paper took a different tactic. The authors wanted double stranded RNA to be produced inside the bees’ bodies. Bees’ bodies (and all bodies) contain all the machinery to produce any type of RNA you want. That’s how viruses work, actually: they force the body to produce the viral RNA. It’s all just building blocks put together in different orders, after all.
So, in order to get it produced inside the bees body, first they designed a plasmid, which is a circular ring of DNA (DNA can produce RNA). This was the thing that they literally just went to the Internet for. They knew the result they wanted to get (the order of the dsRNA), so then they just went online and ordered a plasmid that would produce dsRNA in a certain order, and they got their plasmid in the mail. That’s amazing, right?
Once they had a plasmid, they “transformed” it into S. Alvi, a gut bacteria that’s very common in bees. This is basically like molecular sewing: you snip open S. Alvi‘s DNA, snip open the plasmid to get a single strand, sew the single strand in S. Alvi‘s DNA, and then let S. Alvi sew itself back together again with the plasmid still inside.
Then, getting it into the bees was relatively easy: they dunked the bees in a solution with sugar water and the bacteria. The bees clean each other off, and then they get infected with the bacteria. Now, the next time S. Alvi‘s DNA gets activated in the bee to do normal gut bacteria stuff, it’ll also produce this dsRNA.
From there pretty much everything else was just testing. They tested where the RNA ended up being produced by including green fluorescent protein in their primer, which is a super common (but still cool) tactic in biology. If you include “make this protein that glows green under UV light” light in your plasmid’s instructions, then wherever your RNA is being produced, there will also be bright green light.
They also tested whether dicer and argonaute were active, to see if the dsRNA was actually doing its thing. Finally, they got into whether they could actually make the experiment work. First, they used one kind of dsRNA to interfere with insulin RNA (i.e. disrupt the production of insulin). They found that insulin production halved (or even quartered) in all areas of the bee body compared to control.
As you’d expect, this has pretty dramatic effects on the bees. The bees who had insulin interfered with were more interested in sugar water, and also gained weight compared to normal bees. I’ve put the weight graph down below, as I think it’s convincing. The sugar water graph I’m also going to put down below, but I’ll discuss it later, because it’s kind of weird.
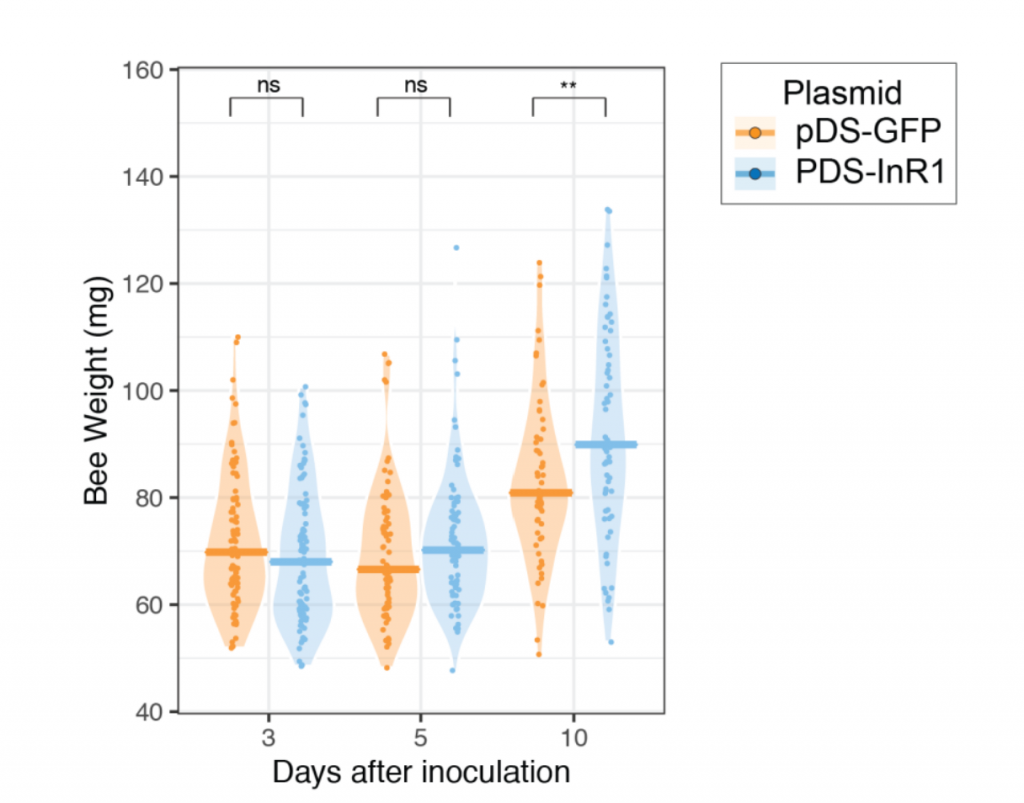
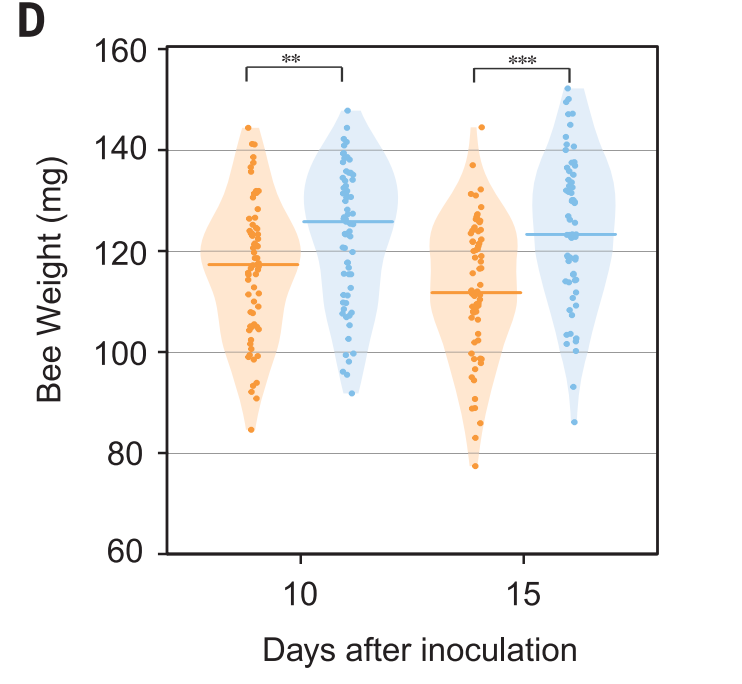
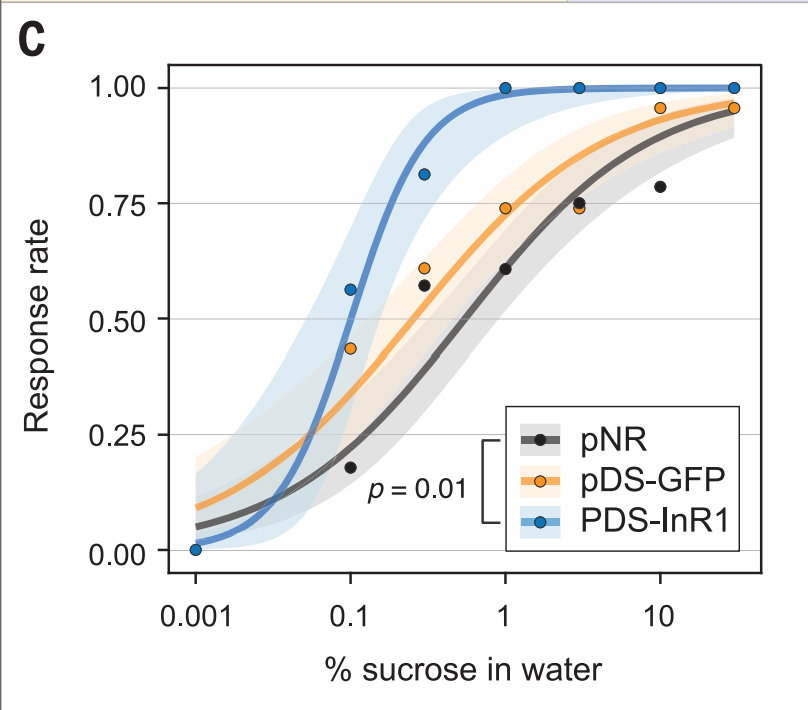
Then, they tried for the interesting stuff. They used another kind of dsRNA to interfere with the reproduction of deformed wing virus (DWV) in bees. The combo of deformed wing virus and Varroa mites are super deadly for bees, and are the main direct causes of colony collapse disorder. So, first, they infected honey bees with DWV, then gave them a plasmid to produce dsRNA to interfere with the reproduction. 45% of infected bees survived 10 days later with the plasmid; only 25% of bees survived without it.
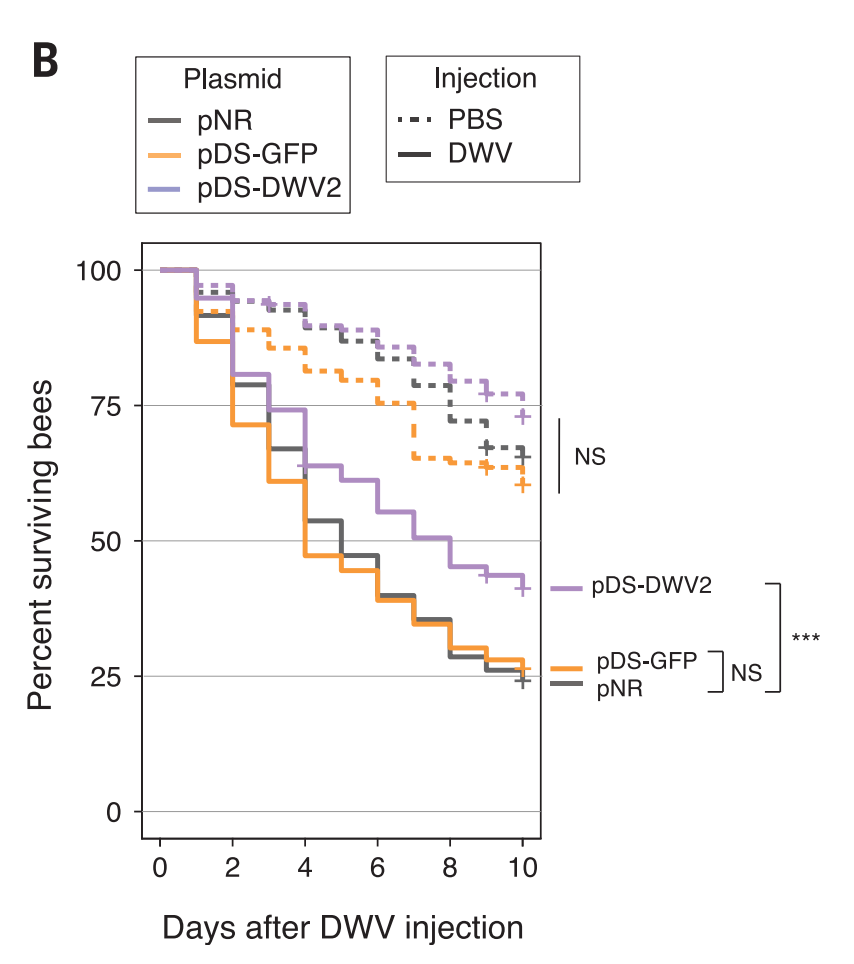
Next, they tried to use dsRNA to kill mites feeding on bees. This is a little more complicated, because the dsRNA gets produced in the bees, and then the mites feed on the bees and ingest the RNA. That then kills the mites. 50% of mites survived after 10 days when they didn’t feed on bees with the plasmid; only 25% of mites survived after 10 days when they fed on bees with the plasmid.
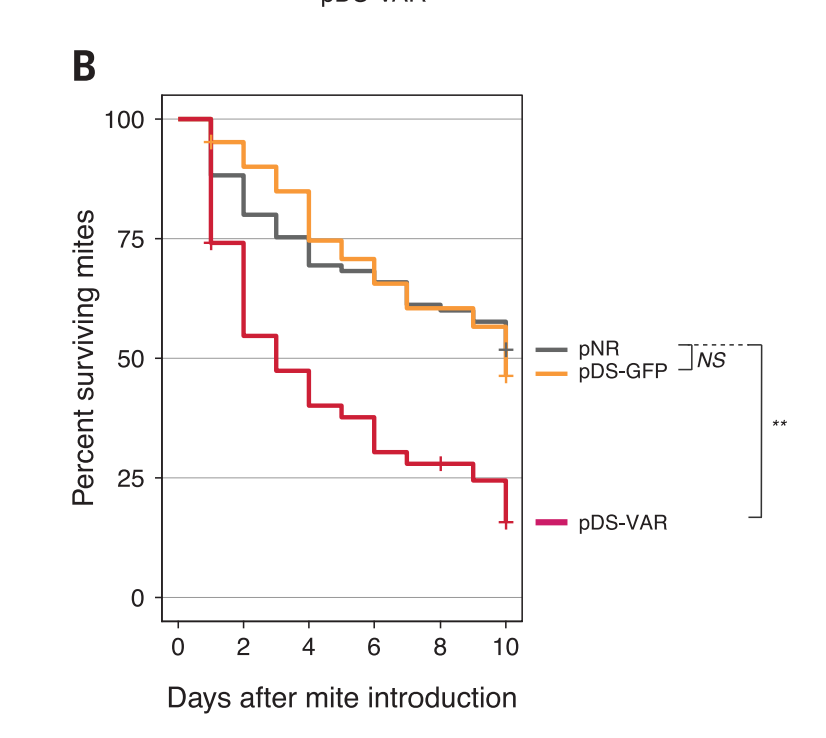
Overall, this is a pretty cool paper with some cool methods. Unless they really, really screwed up their data, it seems like they definitely found an effective way to protect bees against viruses and mites through genetic engineering. A lot more bees survived and a lot more mites died than the control.
But I want to talk about some of the limitations and problems I have with the study, too.
First of all, one of their claims is that, even though they produced dsRNA only in the gut, it was effective throughout the bees’ entire body. They used this graph to show this.
You see that, by day 15, there’s like 10,000 copies of GFP RNA in the gut, 100 in the abdomen, and like 10 in the head for the treatment group. There’s also around 10 copies of GFP RNA in the gut and abdomen for the control group, which is presumably some kind of cross contamination or measurement error.
That’s fine, but I’m not a huge fan of 10 copies of GFP being an error in the control group, but a positive signal in the head treatment group. They try to defend it by the head control group having zero GFP, but I’m not sure that’s the right comparison to make.
I think that the rest of the biomarkers in the head bear that out. Almost all of them are NS (not significant), and I’d imagine the one that is significant is just chance.
So, I’m not convinced any of this stuff makes it to the bees’ head. I think it definitely makes it to the bees’ abdomens, but the effect there is still weird. Look at the biomarker graphs below:
I’ve drawn in a bunch of lines just to point out how confusing the biomarker patterns are. For example, the graphs in row B are for dicer, which should go up with dsRNA (the body produces more of it because it has more dsRNA to dice). Note the y axis is the change, rather than an absolute number (annoying choice on their part).
For both the gut and abdomen, it increases from day 5 to day 10 (column a to column b). However, it stays flat in the abdomen in day 15, but still goes up in the gut. This is supposedly one of their significant results, but what gives? Is there some natural limit in the abdomen that doesn’t exist in the gut?
They buried all these biomarker graphs in the supplement, but I think they’re interesting. At the very least, they complicate the story.
The next issue I have is with the sucrose response graph, which I’ve reproduced below.
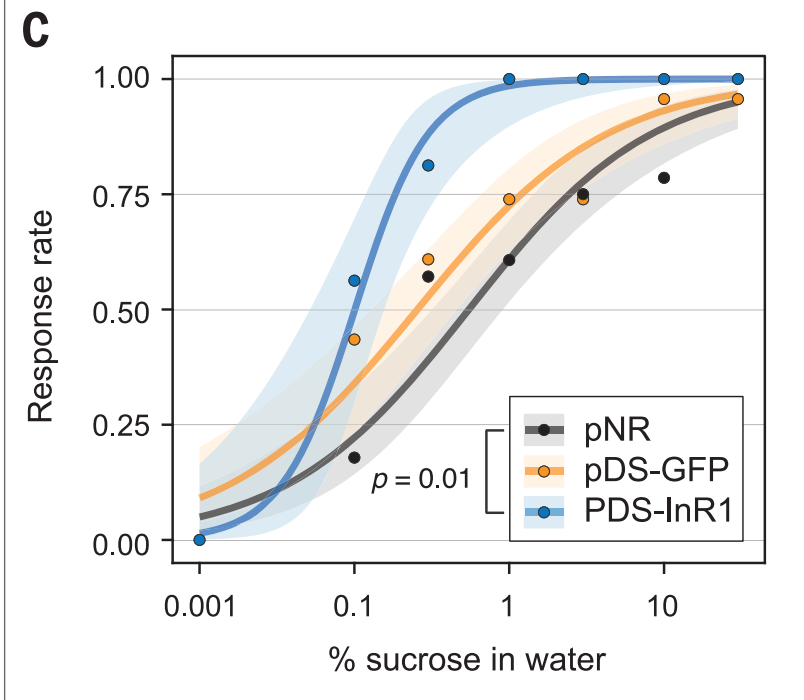
If we just had the pDS-InR1 and pNR, I think it’d be a relatively clear story of insulin getting knocked out and bees becoming more sensitive to sugar. But, it’s really confusing what’s going on with the pDS-GFP bees.
It looks like those bees are consistently more responsive to sugar than the pNR bees, even though they should be virtually identical. Why is that? I really wish we could have seen the weight of the pNR bees compared to the other two, so we could have another point of comparison.
The final issue I have is with the bee and mite mortality rate graphs. Below is the bees with virus graph again.
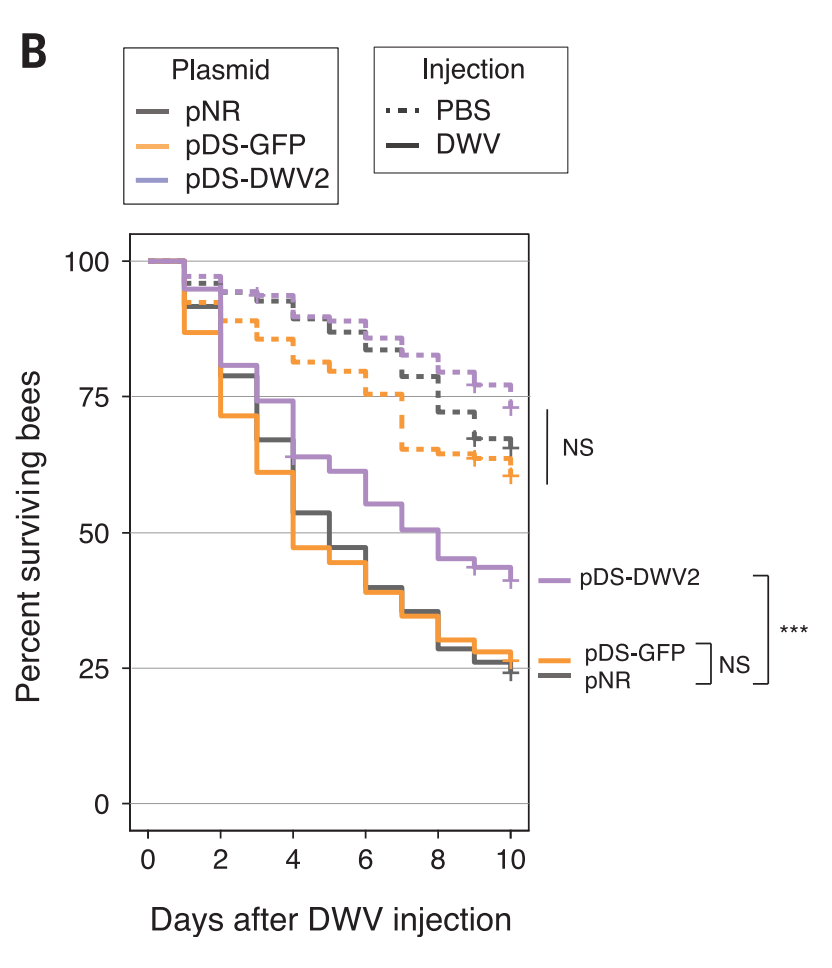
The bees with the protective dsRNA (pDS-DWV) definitely do better than than the bees without (i.e. the bees with just GFP or just the empty plasmid NR) in the treatment group.
But the pDS-DWV bees also definitely do better in the control group, which shouldn’t happen (they’re not being attacked by the virus). The graph says the gap is not significant, and it might be right, but it’s still a big gap. It’s almost the same size as the treatment gap.
I’m also wondering why up to 40% of bees are dying in 10 days in the control group (the orange dashed line). Bees, according to Google, live 122 to 152 days, so they shouldn’t be dying that quickly. I mean, obviously it’s traumatic to get stabbed in the chest with a comparatively giant needle and pumped full of fluid, but, if that’s the case, what effect is that having on the treatment group? How much of the death is from the virus vs. the trauma? Couldn’t they find a better way of infecting them?
I also wonder about the Varroa mite graph, which I’ve reproduced again below. In the graph, 50% of mites die after 10 days in the control group. According to Google, mites live for 2 months. Why are so many of them dying after 10 days?
I’d like to see a control group of mites feeding on bees in “the wild” (i.e. in a normal beehive), to see what the normal survival rate should be.
So, I think these are some strong results, but they’re complicated.
I don’t think the story of how dsRNA moves around the body is super clear, and I think it probably doesn’t make it to the head at all, contrary to what the paper claims.
I think that either insulin is more complicated in bees than this paper assumes (i.e. it doesn’t have such a clear relationship to propensity towards sugar water), or there was something wrong with the GFP bees.
Finally, I think this method does protect against mites and deformed wing virus, but how much it does is complicated by the fact that the bees and mites died a lot regardless of what was done.
Final question: how close is this to production?
Well, barring legal issues, I think this could actually be close. Here are the two big issues standing in the way:
First, it seems like each bee has to be individually treated. The study authors actually tried to see if bees could infect each other with the genetically engineered bacteria, but it only worked on 4/12 newly emerged workers (which is obviously not a large sample size). They’d need to figure out some way to encourage bees to infect each other more, or beekeepers would have to individually treat each bee (which is labor intensive and probably traumatizing for the bees).
The other issue would be with regards to creating the plasmids, putting it into bacteria, then infecting the bees with the bacteria. That’s all expensive and labor intensive, and definitely not the sort of thing that beekeepers would want to do themselves. I’m actually very curious about the total cost of the plasmids for this experiment, and how much that would increase given the number of plasmids you’d need for all the bacteria.
Of course, the ultimate dream would be to do this to humans. Given that there are some viruses (which shall not be named) which currently don’t have effective treatments, the possibility of simply injecting ourselves with gut bacteria of our own transformed to produce dsRNA is really attractive.
I think there are some serious issues with that though. Humans have a really complex immune system compared to bees, and I’m not sure how our immune system would react to a bunch of random snippets of RNA floating around our blood stream. As a last ditch effort in a severe case though… might be interesting. I’ll explore that with my next post.